Introduction
Before virtualization support was available on CPUs, virtual machines were emulating most of the code that a guest OS would run. With VMX, the CPU handles the virtualization. If you are familiar with the hardware context-switches that were available on ia32, it's a bit similar. The hypervisor manages many VMs. When executing code of a VM, there is a hardware context switch that occurs. The context switch saves the current CPU state (but not the general purpose registers) and loads another state that would be the one of the VM. Then code can execute from there. So the VM ends up running "bare-metal". The CPU has changed it's entire context and runs your VM just as it would if the guest was running bare-metal. With one exception: Some operations causes VMExits. For example, when an interrupt occurs, the CPU automatically switches back to the hypervisor context (what they call the VMM). The VMM can then handle the interrupt and change the Guest's state data so that on the next entry it will think it has an interrupt to handle (or not). This is basically how you would handle the timer interrupt on the guest and on the host so that multithreading works. VMExits occur for many other reasons. You could have a VMExit occur when the VM tries to access unmapped memory, when executing "hlt" or many other reasons.
For other articles about my OS:
Networking in my OS
virtio driver implementation
Process Context ID and the TLB
Thread management in my hobby OS
Enabling Multi-Processors in my hobby OS
Memory Paging
AVX/SSE and context switching
Stack frame and the red zone (x86_64)
To view the full source code of my OS: https://github.com/pdumais/OperatingSystem
Goal
The virtual environment we'll be setting up is for a 16bit real mode VM because that's how a real CPU would start. Control will initially be transfered to address 0x00000000. Normally, an entry point to BIOS code should be located there and then the BIOS would eventually load the first sector of the primary HDD and transfer control to 0x7c00. But in my case, I am not writting a BIOS, so only test code will be executed in the VM.
Memory
In a VMX environment, there exists several layers of memory:
- Guest virtual memory: The guest's virtual memory if paging is enabled in the Guest. That memory translates to guest physical memory using the guest's paging structures, entirely controlled by the guest.
- Guest physical memory: This is the physical memory from the VM's point of view. From the hypervisor's point of view, this is just virtual memory that has to be mapped to physical memory. This memory would be allocated lazily as the VM uses more and more memory. This memory is mapped to physical memory by the hypervisor.
- Physical memory: This is the real hardware physical memory as seen by the host's CPU.
For more details about how paging works, please refer to Memory Paging
For the VM to have memory, some of the host's physical memory must be assigned to the VM. The guest's physical memory is seen as virtual memory by the host. To assign memory to the guest, a feature provided by the CPU called "EPT" can be used. EPT works Just like the regular paging system. It is a way to map guest-physical addresses to real physical addresses using a paging structure with a PML4 table on top.
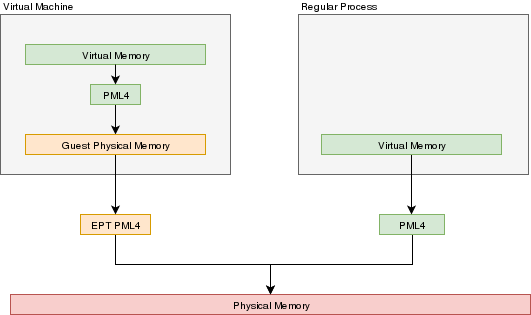
So a guest would prepare it's own internal paging system and have it's own PML4 table. When an a virtual address inside the VM needs to be resolved, it will be resolved to the guest's physical address using its PML4 table. Then, the guest-physical address will be resolved using the EPT's PML4 table. So 2 translations would be done.
EPT is enabled by setting the "Enable EPT" bit in the VM-Execution controls. When enabled, an EPT PML4 structure must be prepared and and the base address of that structure must be stored in the VMCS EPT pointer
Lazy allocation of guest physical memory
When a guest is launched with, for example, 16gb of ram, it would not be desirable to reserve that entire memory on the hypervisor immediately. Instead, it would be preferable to allocate that memory in a lazy allocation fashion. A guest might never use it's entire RAM so reserving it on the hypervisor would be a waste.
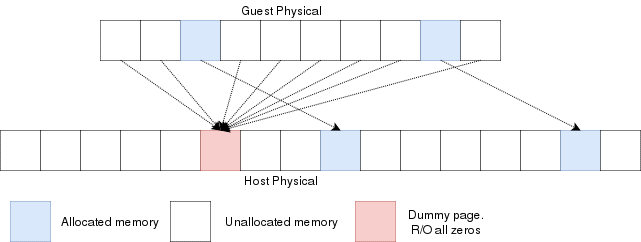
Reading an unallocated guest physical page will return zeros. Writing to it will trigger a EPT violation. The VMM can then reserve a physical page and map it there instead of the dummy page.
When the guest is launched, a single page of write-protected physical memory (filled with zeros) should be mapped to all the guest physical memory. If the VM's BIOS starts probing memory by reading all of it, every 4k would be mapped to that single page so the BIOS would read zeros and think it is reading valid memory. If the BIOS (or the guest OS) writes into memory, a VMexit would occur because writing to any address would map to this write-protected physical page. The hypervisor can then allocate a new R/W page to the EPT's paging structure for the guest physical memory. Of course, if the guest does a write/read-back kind of algorithm the probe the memory, then all the guest physical memory will have been allocated because all pages will have been written to, so all bets are off.
Reclaiming freed-up memory is a different story. The only way the hypervisor can know about memory that can be reclaimed is by using memory balooning. The virtio specification describes a memory baloon device that can be implemented for that purpose.
When the guest will access guest-physical memory that is unmapped to physical memory, a VMExit will occur. The hypervisor needs to evaluate if the guest-physical address falls into the possible addressing space of the VM (i.e. if it is trying to access memory beyond the last byte of the virtual RAM). If the memory is unmapped because of the lazy-loading scheme, then a new page can be allocated. If it is because that memory address should not be mapped, then an exception will be injected back in the VM and the guest will need to handle that.
Translation Lookaside Buffer (TLB)
When the process translate a virtual address to a physical address, the translation is cached in the TLB so that it wont have to walk through the page tables again next time. In a virtual environment, the CPU only has one TLB in which is caches translations for:
- All host virtual/physical mappings
- All guest-physical/physical mappings (of all VMs)
- All guest-virtual/guest-physical mappings (of all VMs).
This could create collisions in the TLB. For example, 2 VMs could have a mapping of virtual address 0x00000000. A simpler case would be two processes with different virtual memory mappings running on the host. For this reason, as I described in another article (Process Context ID and the TLB), the TLB supports processID-tagging. With VMX, two new taggings exist: VPID and EP4TA.
VPID is the tagging of a TLB entry for a specific virtual CPU. This is only tru if VPID is enabled in the VMCS and if a non-zero VPID has been set in the VMCS. In such a case, every guest-virtual to guest physical translations that are inserted in the TLB will be tagged with the current VPID. This means that another VCPU can insert a similar entry in the TLB and it will be tagged with a different VPID. When the MMU looks up a mapping in the TLB, it will only consider those tagged with the current VPID.
EP4TA tagging is done when EPT is enabled. Every guest-virtual to host-physical (going through EPT tables) are cached in the TLB with a tagging of the current EPT,PCID and VPID. The EPT ID (EP4TA) is derived from the current EPTP, so there is no specific ID to set, unlike PCID and VPID.
If VPID is not enabled, then the VPID tag is always 0 and is always ignored during lookups. A lookup while EPT is active (during non-root operation) will only consider entries with a matching EP4TA.
I'm a bit confused by what the Intel documentation says about EPT and the TLB. From what I can understand, it seems like the TLB will cache guest-virtual mappings to host-physical addresses. Those are called "combined mappings" When EPT is enabled, these entries will be tagged with the current EP4TA,VPID,PCID. If VPID is not enabled:
- entries will be tagged with VPID=0.
- On vmexit/vmentry, all entries with VPID==0 will be invalidated, regardless of EP4TA tag.
For this reason, I am enabling VPID with a tag of "1" for all my VCPUs. The documentation clearly states that having the same VPID for different EPT is acceptable.
When the guest will invoke "invlpg" or "invpcid", these instructions should be trapped because they will invalidate all requested TLB entries regardless of the current EP4TA. So the VMM should set a VMExit on those operations and emulate them using "invept. That's what I am understanding from section 28.3.3.1, but section 28.3.3.3 says that when EPT is enabled, there is no need to emulate these instruction.
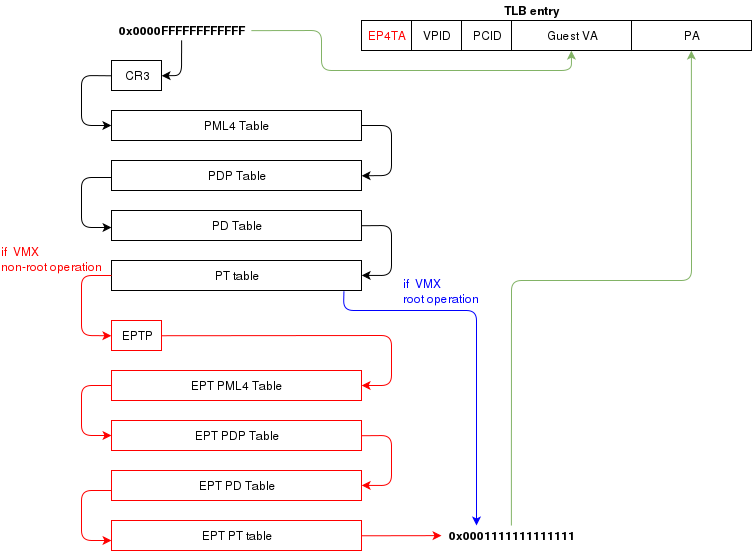
When to invalidate
When a VMExit occurs because of an EPT violation, the faulting page will always be invalidated in the TLB. In my OS, I use the EPT violation handler to allocate more memory to the VM. When the VM resumes, the TLB will be up to date the VM will be writing data in the correct physical page. If the VM is rescheduled on another core where the stale TLB entry exists for that address, then it will be accessing the wrong physical page. The TLB was only invalidated on the CPU that detected the EPT violation. For this reason, the host needs to do a TLB shootdown on all host CPUs. This done by sending a IPI to all other CPUS. The CPUS will then execute "invept" to invalidate TLB entries associated with the EPT in question.
The "invept" can be used to invalidate ALL tlb entries tagged with a specific EPT, or all EPT.
Initialization
The initialization of vmx mode is well described. There are a couple of web sites with examples that can be found using a simplegoogle search. But the Intel SDM #3 describes an algorithm to do so. Basically, this is what needs to happen:
- detect support for VMX
- create a VMCS for vmxon
- execute vmxon
- create a VMCS for a VM and write all required data in it
- run vmlaunch
It's important to note that VMXON should be executed on all CPUs and this should be done when the hypervisor starts. In my OS, I do this as soon as I switch to long mode on all cores and I leave it on all the time. The tricky part is to get the VMCS for the guest initialized correctly. I had to spend quite some time fine-tunning the fields. If VMLAUNCH fails, the zero flag will be set, then you can do a VMREAD to VM_INSTRUCTION_ERROR to get the error code. Then, the SDM (chapter 26) describes all the checkings that the CPU does so you can walk through those and make sure your code is compliant.
Also, it seems like access rights and segment limits for guest segment descriptors still need to be properly set even if the VM will run in real mode. If I recall correctly, it's because they will be checked even in real mode and that is how "unreal mode" works.
I am not going in the details about this because everyone talks about that and the SDM has very good documentation about it.
Multi-tasking (in a multi-core environment)
Running a VM in a multitasking environment requires a bit of logic that is beyond that the Intel manuals describe. This is because a VM really is just a process getting a time slice with the scheduler. So when the process is scheduled out, the entire VM is paused.
Every VCPU is managed by one and only one thread in the OS. So each thread has a VMCS (if it is running a VCPU) Before the task get's scheduled out, vmclear is executed so that any cached data gets flushed in the VMCS. At least that's what Intel recommends to do. When a task is scheduled back in, VMPTRLD is executed to load the VMCS (if any) that this task manages.
When a timer interrupt occurs and it's time to do a context switch on the host, since the VM runs as part of a process on the host, it is normal that it gets interrupted and that time is given to another process. That process might want to run a VM also, or maybe it's just a normal application. When the timer interrupt occurs, this will trigger a VMExit and the CPU will be back at executing code in the context of the host. The thread will change. When getting back to this thread, we will do a VMRESUME to continue executing the VM. We will also reload the VMCS with VMPTRLD because another process might have loaded another VMCS. We could even be running on another processor in a multi-core system. And remember, loading a VMCS is only loaded on the current core. So with 4 cores, you could have 4 different VMs running
When the VMM is running in root-operations because of a VMexit, it could have been scheduled out at any moment before doing the VMRESUME. So when VMRESUME is executed, it is possible that VMCLEAR was executed before because of a task switch. For this reason, the OS checks if VMRESUME fails and executes VMLAUNCH instead.
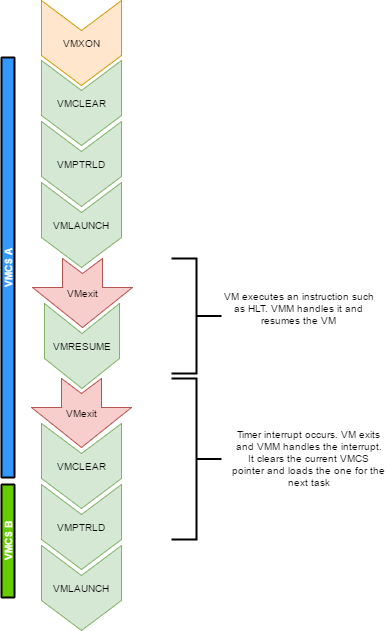
The OS should avoid scheduling VCPUs from cores to cores because moving a VCPU to another core makes it mandatory to do a VMCLEAR. My OS does not make such effort and blindly does a VMCLEAR on every task switch. So performance could be improved there.
And interesting point here is that after executing VMLAUNCH, control never returns to the calling function (unless vmlaunch fails). VMLAUNCH and VMRESUME will jump in the guest's code without pushing a return address. A VMExit handler has been defined in the VMCS, so from the host's point of view. control skips from vmlaunch to the handler. Also, since the stack is setup in the host's section of the VMCS, the VMM gets a brand new stack everytime the VMExit handler gets called. This means that there is no need to pop the stack before executing VMLAUNCH/VMRESUME
VM Exits
When the cpu is running in non-root operation (running VM code), it's pretty much as if the VM was running bare-metal. But Several events can make the VM exit back to root-operation (the hypervisor). A VM exit will restore the CPU state back to what it was set in the VMCS before vmlaunch. The hypervisor must determine what caused the VMExit and take proper action. VMExits are similar to interrupts because the general purpose registers are not automatically saved. A VMExit handler must save the registers and restore them before resuming the VM otherwise the VM will be corrupted. As per the host section of the VMCS though, a separate stack is being set. The VMM could alter the VM state by changing data in the VMCS before resuming the VM. For example, if the VMExit occured because of an exception of type #UD, the VMM could emulate the invalid instruction and change general purpose registers and flags in the VM.
Interrupts
When an interrupt needs to be delivered to the host, a VMExit will occur and control will be immediately given to the VMM's exit handler. At that moment interrupts will be disabled (flags.IF=0). When bit 15 (Acknowledge interrupt on exit) is cleared on the VM Exit control, the interrupt is not acknowleged by the processor. This means that as soon as the "IF" flag becomes 1 (ie, when executing "sti"), the interrupt handler will get called.
In my hypervisor, if such a VM exit is detected, the kernel executes "sti" and finally "vmresume". This will let the host execute the handler as soon as interrupts are re-enabled, then control will be handed back to the VMM exit handler to execute "vmresume"
It is worth noting that it doesn't seem to matter if interrupts were cleared before VMLAUNCH. Interrupts will still trigger a VMExit and the interrupt will be pending until the VMM enables them (using "sti")
Multiple VCPU
supporting multiple VCPUs for a VM requires having 1 VMCS per VCPU (just as if they were all used in independent VMs). But the hypervisor has to make those CPUs dormant until a "Startup IPI" is sent. From there, the hypervisor can configure the VCPU to start executing code at the desired address. But the main thing here is that only one VCPU would be started when the VM is launched. My OS does not yet support this. I am focussing on 1 VCPU for now
One thing to consider when running multiple VCPUs is that one of them could trigger a VMExit because of a memory allocation requirement (as described in a previous section of this article). In such a case, another VCPU could be running, or the current thread could get scheduled out with another VCPU one being scheduled in. So extra care should be taken to ensure that memory does not get corrupted.
Other thoughts
There are many other things to consider to improve performances and to make the design robust Things to consider are:
- Trapping "hlt" with a vmexit so we can schedule out an idle VCPU
- Trapping TLB/MMU operations to better manage memory
- Trapping IO operations so that the hypervisor can retain control over hardware.
- Setup a virtual apic so that VCPUs can receive interrupts (and make a scheduler).
The code
For reference, this is a part of my VMX code:
What next?
There is still some work to be done for implementing a full hypervisor. The work done here only creates virtual CPUs. The hypervisor needs to emulate other hardware such as a hard drive, a network card, a video card, keyboard etc. I would probably write virtio devices since they are easy to code and very efficient. Another big part that is missing is a BIOS. The hypervisor has to provide a BIOS for your guests since they rely on that to boot.